The transformation of carbon dioxide (CO2) into carbonaceous molecules for the chemical industry (e.g. alcohols) and fuels (e.g. hydrocarbons such as alkenes or alkanes) is a strategy that is increasingly being developed in academic and industrial laboratories. Indeed, freeing ourselves from traditional fossil carbon sources, and developing chemical synthesis processes based on new carbon sources, is a response to the climate crisis.
CO2 from the atmosphere, or more certainly that captured in the major industrial centers that emit this gas (refineries, cement plants, thermal power stations, etc.), can be one of the carbon precursors that will enable us to move towards a greener chemistry. And if the energy used forCO2 transformation reactions is renewable and intermittent, such processes have the advantage of enabling this energy to be stored in a sustainable chemical form. For example, the transformations discussed in this publication can use "solar" electrical energy to power an electrolyzer whereCO2 is converted (by electroreduction) into various carbonaceous products. These electrolyzers use electrical energy to oxidize water (producing oxygen and hydrogen ions) at one electrode (anode) and reduce carbon dioxide at the other (cathode), where hydrogen ions are also reduced to hydrogen gas.
Due to the high stability ofCO2 and the complexity of the reactions involved in its transformation, the practical implementation of these processes requires the development of efficient, low-cost, stable and selective catalysts. The most promising metal for tomorrow's electrocatalysts is copper, but it still suffers from major shortcomings: insufficient efficiency and low selectivity. In particular, the reduction of water used as a "green" solvent in these devices competes with the reduction ofCO2, as water is more efficiently converted into hydrogen. This competition leads to yields of carbonaceous products that are too low to be profitable.
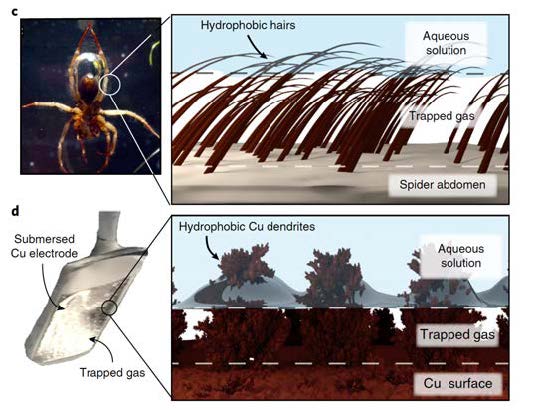
Faceplate effect. Use of a hydrophobic surface to trap a layer of gas at the solution-solid interface. This is illustrated by the underwater respiration of a diving spider (top left) and a hydrophobic dendritic copper surface acting as a catalyst for the electroreduction of aqueousCO2 (bottom right). The spider photo is adapted from Seymour and Hetz, with permission from The Company of Biologists Foundation.
The team led by Marc Fontecave, Professor at the Collège de France and holder of the Chemistry of Biological Processes Chair, and Victor Mougel, CNRS research fellow, has just developed a new strategy for making copper-based catalytic materials much more selective for the reduction ofCO2 to hydrocarbons. Part of the strategy's originality lies in the fact that it is inspired by a natural strategy developed by aquatic spiders to maintain oxygen bubbles underwater, on the surface of their abdomen and legs, enabling them to breathe in the water. To do this, they use the "plastron effect", which takes advantage of the surface hairiness of their legs and abdomens, which are highly hydrophobic, thus repelling water and effectively retaining oxygen bubbles.
Considering that one of the keys to improving the selectivity of copper-based catalysts lay in the accumulation ofCO2 gas on the one hand, and the elimination of water from the surface of the material on the other, the researchers were inspired by the "breastplate effect" described above. By simply attaching a layer of hydrophobic threads (in this case alkyl chains) to this surface, which in a way mimic spider hairs, they have radically changed the selectivity of this catalyst, favoring the transformation ofCO2 to the detriment of water reduction. The result is impressive: while the unmodified catalyst produces ethylene in a low yield of 9% and ethanol in a yield of 4%, the modified catalyst produces ethylene in a yield of 56% and ethanol in a yield of 17%, accompanied by a drastic drop in hydrogen yield. Ethylene and ethanol are particularly interesting products for the chemical industry. Ethylene is the precursor of a large number of polymers, while ethanol is both an industrial commodity and a fuel. On a more fundamental level, this work clearly demonstrates for the first time the importance of the hydrophobicity of catalytic material surfaces for the selective transformation ofCO2. It opens up new prospects for optimizing catalysts for tomorrow's electrolyzers.
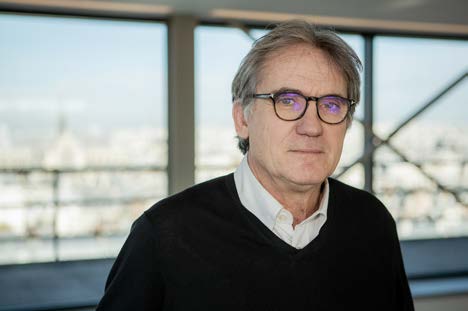
Prof. Marc Fontecave holds the Chemistry of Biological Processes Chair at the Collège de France.
He is Director of the Chemistry of Biological Processes Laboratory (CNRS/Collège de France) and was awarded the CNRS Silver Medal in 2004.