Diatoms are unicellular photosynthetic micro-algae that live in both fresh and salt water. There are over 250 genera and more than 200,000 species. Diatoms have a shell called a frustule, made of amorphous silica. These frustules feature complex 3D architectures obtained by genetically controlled self-assembly of regularly arranged nanometric elements (pores, channels, protuberances).
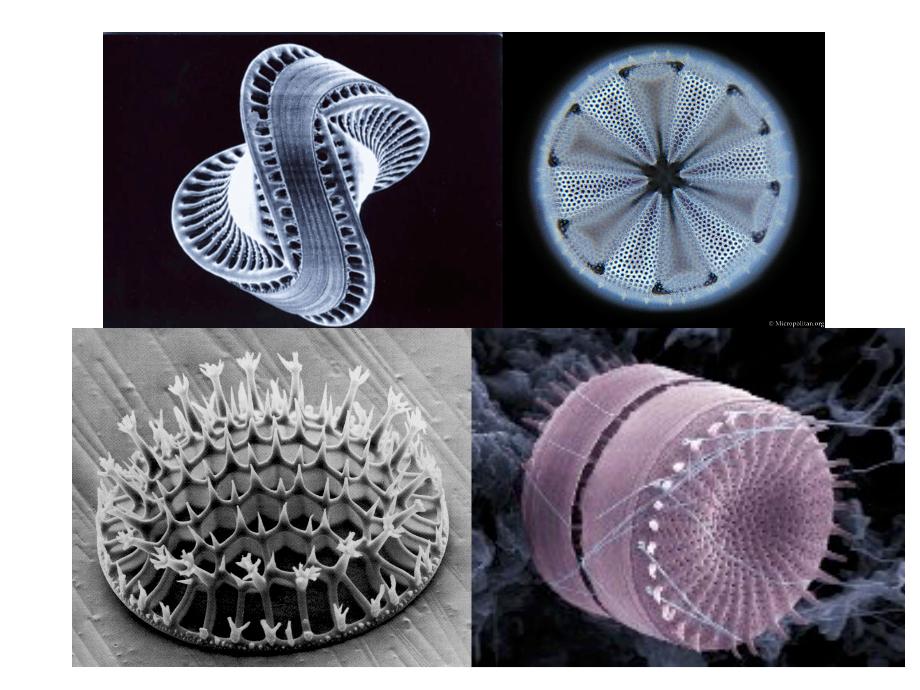
These highly aesthetic and functional structures have inspired nano-artists as well as architects and engineers in the design of buildings and aircraft. Using photosynthesis as their energy source (photo-assisted conversion of carbon dioxide and water into sugars and oxygen), diatoms live where sunlight can penetrate. In other words, in the euphotic zone between the surface of the sea or lake and depths of around 200m. They are essential to life on earth, producing around 20% of the oxygen we breathe. When they die, they settle to the bottom of the ocean, producing an immense carbon dioxide sink. Their role is therefore essential in the earth's carbon cycle.
These microalgae are also rich models for the study of silica biomineralization processes. The fact that the genomes of several diatoms have been sequenced in the last decade, combined with advances in physico-chemical characterization methods, has clarified in part the relationships between the biomacromolecules present, self-assembly processes and the construction of complex silica architectures. Three groups of biomolecules are strongly associated with the biosilification process: silaffins, silacidins and long-chain polyamines. Silaffins and silacidins are peptides or proteins bearing numerous phosphate residues attached to amino acids (serine and threonine), while long-chain polyamines are essentially linear non-protein components made up of oligo-propyleneimine chains. Through self-assembly processes, mainly under electrostatic control, these biological macromolecules form bio-aggregates. At first order, the size of the bio-aggregates and the amount of silicon oligomers bound to them determine the final size of the silica spheres in the diatom's silica architecture. In vitro, these biomolecules also have the capacity to considerably influence the kinetics and structure of the silicas formed. Understanding these mechanisms is a source of inspiration for developing new synthetic processes and original organic-inorganic hybrid structures. From the point of view of fundamental research, diatoms are likely to contribute to the resolution of one of the still unresolved questions in biology: What is the detailed involvement of the genome in the creation of forms, and what are the levers of control over their evolution? Although a few competing hypotheses have recently been put forward for a small group of diatoms, we must be wary of jumping to hasty conclusions. More observations and intracellular physico-chemical analyses are needed to better understand the nature and dynamics of the phenomena involved.
In terms of materials science, diatoms open up new avenues for research and development. The highly porous architectures of their frustules have made it possible to use diatomites (fossil diatoms) as: adsorbers of toxic molecules and heavy metals, carriers of reactive molecules and catalysts, filters and membranes, vectors of active ingredients in agriculture and cosmetics. Because of their abundance and the structural evolution of their frustules in response to pollution, diatoms are also used as indicators of water quality. Since diatoms can be produced by aquaculture and present a wide diversity of nano-, micro-structures and morphologies, they are an easily accessible and sufficiently abundant source to obtain the quantities required for materials science studies.
In particular, over the last ten years, since the explosion of nanotechnologies, a great deal of research has been carried out into the multi-scale porous structures of diatoms. The potential applications of these new diatom-based materials or devices were extensively presented and discussed in the sixth lesson. Chemical and/or biochemical modification of diatom frustules leads to materials with a range of original properties. Porous silica frustules accept high levels of active ingredients, are biocompatible and easily functionalized with organic or biological components, enabling the development of hybrid therapeutic vectors for controlled drug delivery. Theranostic vectors that combine targeting, diagnostics and therapy have already been developed. Recent studies have also demonstrated that these microalgae can be a sustainable source of biofuels. The nanopore structures of frustules are similar to those of photonic crystals, in which photons propagate like electrons in a semiconductor crystal, generating zones of permitted and forbidden energy. Thanks to this property, frustules can be used as micro-lenses to optimize optical responses and concentrate light in energy conversion and storage devices such as photovoltaic cells, dye-sensitized solar cells, photoelectrochemical cells, photo-rechargeable batteries... On the other hand, the topotactic transformation of diatoms into various materials: semiconductors, conductors, piezoelectrics (TiO2, BaTiO3, Si, diatom-graphene nanocomposites, etc.) opens up a host of possibilities for the development of new materials.) opens up a wide range of possibilities for the development of materials with properties that can be applied in the energy and environmental fields. In particular, the photoluminescence or conduction properties of these materials are sensitive to the adsorption of gases, molecules or biomolecules. This sensitivity, amplified by the multi-scale porosity of frustules, makes it possible to develop high-performance gas sensors and biosensors. The transformation of silica frustules into the element silicon enables the development of Li-ion batteries with negative silicon electrodes, whose performance is promising.
Diatoms can also form stable colloidal suspensions, enabling the lithography of microelectronic circuit components and sensor arrays. These components can be chemically modified in a second step by ALD(Atomic Layer Deposition) , further diversifying the functionalities available.
Undoubtedly, a better understanding of diatom structure and genomics, their mechanical, optical and photonic properties, and their bio-mineralization process will lead to the nano-fabrication and engineering of new materials and devices. Diatoms will continue to play an important role in biology and materials science, as their beauty continues to inspire all who study them.