We work at the frontiers of the unknown
A specialist in soft matter and fluid mechanics, Lydéric Bocquet studies nanofluidics and its special properties with a view to finding practical applications for the energy transition.
He has been invited to join the annual Technological Innovation Liliane Bettencourt Chair for the year 2022-2023.
Nanofluidics is the study of fluid behavior in nanoscale channels. How did you become interested in this transdisciplinary science ?
Lydéric Bocquet :I was originally trained as a theoretician. I studied statistical physics, i.e. physical systems made up of a large number of particles. Quite early in my career, I became interested in confined fluids and their particular properties, rather from a theoretical angle. But I realized that the question of fluids on very small scales was singularly lacking in well-controlled experimental studies. Many phenomena can be predicted theoretically, but without the means to apprehend nature's reality (which is often far more unexpected than we could have hoped), this remains somewhat futile. The major stumbling block was that we didn't know how to build nanosystems suitable for studying fluid transport at nano-scales, let alone quantify the molecular flows within them. In 2002, when I became a professor at Lyon University 1, I decided to make a switch in my research by developing my own experimental activity. With a few colleagues, I set up a group focusing on what is known as " soft matter ", and then turned to the field of fluid transport in very small systems. Together with my team, I set out to explore fluid flow in nanosystems, such as nanotubes, or more recently graphene[1] ; all these systems have opened up the field of possibilities for tackling these questions. Previously, the few results in this field had focused on membranes, and many of them had been very surprising or even controversial, for example with regard to the properties of nanotube membranes for water transport. Between 2008 and 2010, together with a few colleagues and some courageous students, we set out to investigate this problem by studying flows in single nanotubes. That was the start of the adventure, and since then I've been ploughing my furrow around what is known as " nanofluidics " - the general study of fluid properties at nanoscales. I have tried to understand emergent properties, which differ from what is usually known at large scales, which has made it a disciplinary field in its own right, at the interface between the continuums of hydrodynamics and the molecular, even quantum, nature of condensed matter. We work at the frontiers of the unknown, and some of our discoveries are frankly unexpected.
You recently worked on a so-called " ion neuron ". What is this ?
This is typically an unexpected application of nanofluidics. It began with a theoretical study of the properties of ionic systems in two-dimensional systems. We have recently been able to fabricate such channels using what we call " van der Waals assemblies ", developed by the team of Nobel Prize winner Andre Geim, with whom we collaborate extensively. These assemblies are very interesting, as they enable the fabrication of extremely fine channels. So, for the first time , we were able to realize and perfectly control fluid or ion flows through channels measuring just a few angstroms[2], i.e. almost in two dimensions. In general, the properties of two-dimensional systems are very strange ; ions interact very strongly, for example. It had been predicted that assemblies of ions would agglomerate to form a spaghetti-like structure. Through theory and numerical simulations, we have seen that it is possible to break these spaghetti, but that they can then reassemble. It's a very complex dynamic. However, as these ultra-confined ion filaments are of considerable size, this phenomenon takes time to take place ; and we see the appearance of a memory phenomenon. It's a functionality that evokes, even mimics, that of the neurons in our brain. We first demonstrated this theoretically in 2021, by reproducing some very basic neuronal functions. Then, in 2022, we moved from theory to experiment, and demonstrated these mimicked neuronal functions in our two-dimensional channels. In particular, with these nanofluidic channels, we reproduced in a very similar way the functions of the synapses which, in our brain, are the basis of learning. This is a perfect example of a completely unexpected result : we confine water to the nanometric scale and observe behaviors that have nothing to do with what we know on a larger scale.
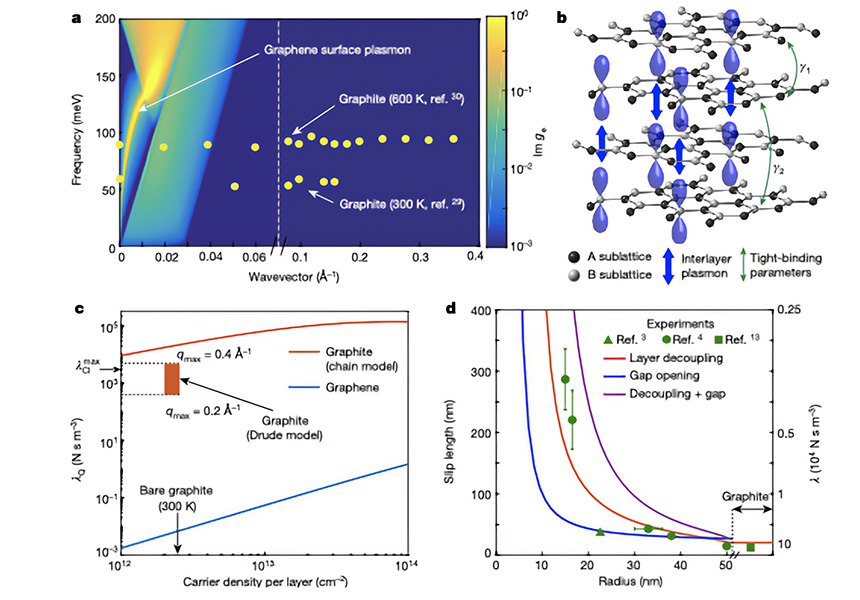
Can these results be applied to artificial intelligence ?
We're still a long way from application, but in theory, yes, a path has been opened up. We hope to be able to follow it. The brain works with ions and water, and has opted for ionic circuitry, which we want to try and mimic. This has not yet been achieved. But we're beginning to see the potential of nanofluidic systems. For the moment, we're working with a single neuron-like system. The idea is to create parallel systems that can communicate with each other, i.e. ionic circuits that can perform ionic calculations. This could be one of the major challenges of this emerging field. Interestingly, there is a real underlying energy issue. Artificial intelligence consumes an enormous amount of energy, while the brain operates with mind-boggling economy (the equivalent of two bananas a day !).
This science has been booming in recent decades. What are its other major challenges today ?
The challenges of nanofluidics are governed by two aspects. The first is that a disciplinary field is being established as a result of a cascade of new phenomena and discoveries, many of which are still unexplained. From a scientific point of view, we need to give ourselves the means to understand these new notions. For example, we now know that quantum phenomena come into play, which was not at all expected, especially in hydrodynamics. This fundamental aspect is very exciting, because we're dealing with completely new notions, and I'm learning every day. The second aspect, which I've been able to experience for myself, is that these nanoscale properties have repercussions not only on various scientific fields, but also on fields of technological application, such as water desalination and osmotic energy. The path from fundamental discoveries to their application in technological fields is a short one. Some of our first experiments, involving boron-nitrogen nanotubes, showed that very strong electric currents could be generated by creating a difference in salinity between very salty water and fresh water. This is what we call " osmotic energy ". We patented the technique and then co-founded a start-up, Sweetch Energy, which now employs over thirty people. The company will be setting up an industrial pilot with the Compagnie Nationale du Rhône (CNR) and EDF in 2023. For me, this is a fine illustration of the impact of fundamental science. There are opportunities for rapid transfer between fundamental science and technological applications, which are in constant interaction.
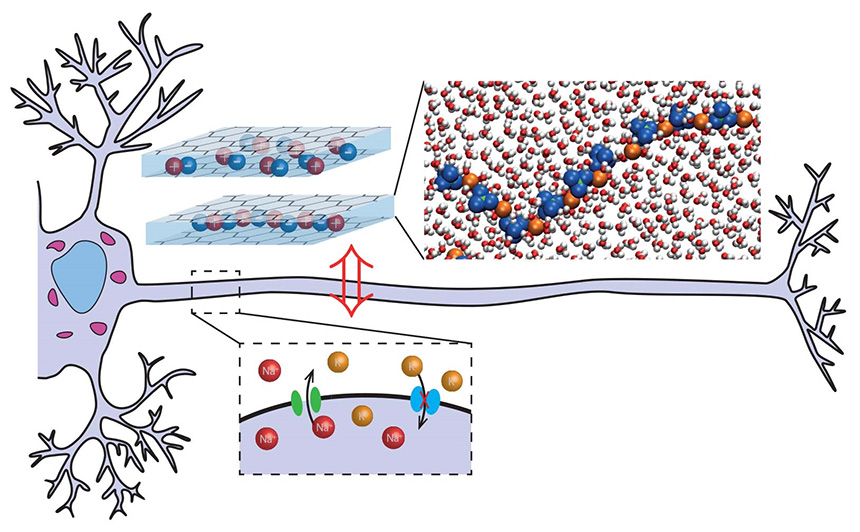
Do these two aspects of your team - basic research and innovation - coexist without the imperatives of one getting in the way of the other ?
This is a point to which we pay close attention. On the one hand, these two aspects are in constant dialogue, as they are components of one and the same team: Micromégas, at the École normale supérieure. However, they do have different imperatives and goals. The sole aim of the fundamental part of the team is to produce knowledge for the advancement of science. The innovation part, on the other hand, aims to file patents, set up industrial contracts and, eventually, develop start-ups. The two sub-groups are therefore very different. Getting them to interact and collaborate is an incredibly rich experience, especially when it comes to seeing the phenomena we observe fundamentally translated into technological innovations in desalination and energy. This remains a daily challenge, as the objectives remain distinct and so do the scales. To achieve successful technology transfer, it's not enough to desalinate water on the scale of a nanotube on the basis of a new phenomenon, however beautiful may be: this phenomenon must be implemented to purify liters, or even cubic metres. It's a completely different vision, and therefore a considerable challenge... but a truly exciting one.
What challenges does a researcher face in developing experimental tools ?
The challenges differ, once again, between the fundamental team and the innovation team. For the former, it's a question of developing new instruments that give access to unprecedented measurements. For example, between 2010 and 2016, we were concerned with measuring what happens inside a single nanotube. As you can imagine, it would take billion years to fill a glass of water with a single nanotube. So we asked ourselves how we could measure the flow of water on this scale. Since we couldn't use standard tools to do this, we had to develop our own approaches. This is quite typical of our work at : we build systems, think up new tools, both experimentally and theoretically. For example, the results we obtained in 2016 were difficult to understand within the usual frameworks so we hypothesized a quantum phenomenon at work. This was rather unexpected, as it's hard to imagine a priori water, a relatively classical substance at room temperature, in a " quantum situation ". So we sketched out a new theoretical framework for understanding what we were observing. I'm also currently developing a project with two other colleagues : one from Cambridge and the other from the Max-Planck Institute in Mainz. Each of them brings his or her own speciality to bear on the development of new common tools that respond to these new theoretical frameworks. On the innovation side, many of the challenges lie in scaling up. Once again, how do you go from a fundamental discovery made at the nanometric scale to a useful application for society ? To do this, we need to be able to make the transition from the single nano-channel of our experiments to a material that can be used to manufacture hundreds of thousands of square meters of membrane. This means reinventing the path, starting from a fundamentally established plan. That's what start-up Sweetch Energy has done. It manufactures membranes from biobased materials on the scale of square meters, reproducing the same phenomena seen in our nanotubes. This scaling-up process is highly complex. The first part is carried out in the laboratory. We go from nanotubes to membranes measuring just a few square centimetres - and there's a world of difference between these two orders of magnitude - and then on to the even larger scale of the device.
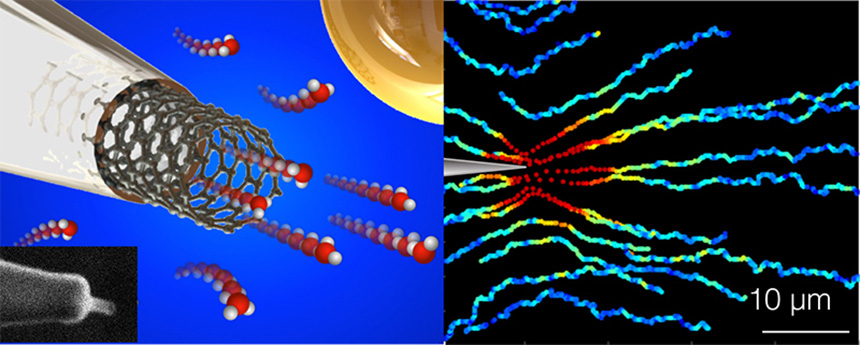
This year, you're a guest researcher at the Collège de France's annual Technological Innovation Liliane Bettencourt Chair. What do you expect from this experience ?
First and foremost, there's the pleasure of teaching, of passing on knowledge, and the excitement of knowing that it can instill in the audience a vocation, a passion, or simply an interest. Through this Chair, I hope to be able to show the general public that French research is fighting to remain at the top scientific level, while being fully aware of the challenges linked to the energy transition. New solutions can emerge. These disciplinary fields and this somewhat relentless and often risky approach that my colleagues and I have been following for the past fifteen years, are all part of this process. The Sweetch Energy start-up and all the applications of our work are rooted in this desire to transform our approaches in line with the climate changes we are experiencing.
You've also taught at the University of Lyon and the École normale supérieure. How important is teaching in the life of a researcher ?
That depends very much on one's sensibilities Personally, I've always loved teaching. I believe that teaching crystallizes thought and catalyzes research. I like to explain to others so that I can learn better myself, and I find that teaching forces us to question ourselves, to explore little-known or uncharted areas. To get out of our comfort zone, in short. That's the very definition of research, and it's something you find in teaching. You're bound to have to teach things you don't know very well, and this aspect of my career has benefited me enormously. In particular, I've published articles that stem directly from my lectures, even articles that are a little anecdotal, as I've worked a lot on everyday physics. For example, I published a paper on the physics of ricochet, which has its origins in an exercise I developed in response to a question from my son, who was eight years old at the time (he's twenty-eight now). I also worked on cooking potatoes, waxing skis and gliding on snow, among other things. I had originally thought of these subjects as teaching material. In the end, they turned out to be particularly interesting research topics. I must say, too, that I've been lucky enough to work with some exceptional PhD students, who are willing to take risks and explore uncharted territory together to advance the discipline. We pass on a lot, but we also receive a lot.
Interview by William Rowe-Pirra
Glossary
[1] Graphene : a two-dimensional material made up of regularly assembled carbon atoms.
[2] Angstrom : an angstrom is a unit of measurement worth 0.1 nanometres, or one tenth of a billionth of a metre. It is used for measurements at the atomic scale.