Interview with Thomas Lecuit
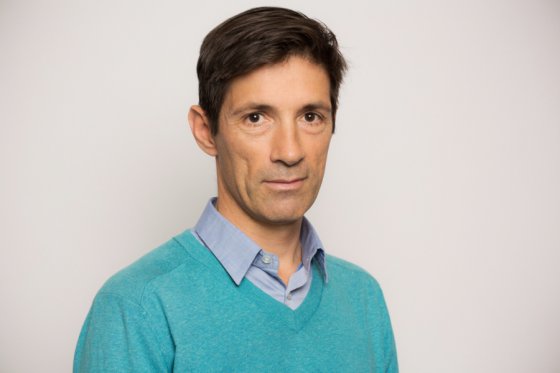
Thomas Lecuit is a biologist. He is interested in the notion of biological information and morphogenesis, i.e. the processes that govern the development of living structures. His work, like his lectures, is a fertile ground of interdisciplinarity where biological, physical and computational sciences mingle.
Since 2016, Thomas Lecuit has held the Dynamics of Living Systems Chair at the Collège de France.
How did your interest in morphogenesis come about ?
Thomas Lecuit :I' ve always been fascinated by the question of emergence. In other words, the emergence of more or less complex structures from something fundamentally simple. An example that appeals to many children, including myself at the time, is that of the butterfly that emerges from the chrysalis. If you were to look inside a developing chrysalis, you'd see nothing but liquid. Faced with this observation, we might ask : what initial information is necessary and sufficient to account for the appearance of the more complex living structure ? How does one go from shapeless liquid to butterfly ? This is a very profound question in the sciences, one that really arises in several areas of physics, and is particularly striking in biology, where it goes back a long way. It was already of interest to Aristotle, gave rise to the first theories of animal development in the days of the ancient Greeks, and has been the common thread running through many theories of living things down the centuries. What's interesting is that, in some respects, it has led us to question the very nature of living things. So for me, this fascination goes back a long way, and it has never ceased to call on a variety of disciplines. In the beginning, my approach to the question was purely genetic or biochemical, but over the years, over the last twenty years, I have also taken into account notions from physics.
You're trying to understand the fundamental paradox of living matter. What is it ?
The paradox lies in the fact that, when we observe living matter, we see a form endowed with apparent stability and permanence. We look in the mirror one day and see the same thing the next. But if we look at the processes that govern us on a cellular and molecular scale, we discover that this apparent permanence conceals a very dynamic : the components of living matter are in constant renewal. Molecular elements can change in tens of seconds, while cellular components can change in minutes, hours or days. Nothing is really stable. This is completely contrary to our intuition of a permanent form. Take the example of the construction of a building : it will be stable, because its components will have been sealed in a stable manner. This is not the case for living things, where permanence goes hand in hand with the ability to adapt to different environments. It's a form of permanence that doesn't depend on the stability of local interactions, but rather coexists with and even depends on permanent dynamics. But then, what ensures the ability to maintain form if the components are in constant renewal ? The study of dynamic systems in physics has shed much light on this point. And this is also where the important concept of information comes into play, to which we'll return later.
How has your work shed light on this paradox ?
First and foremost, our research has brought this paradox to light. Developments in microscopy over the last few decades have enabled us to characterize morphogenetic processes and dynamics on a molecular and cellular scale. The starting point for our research is what we already knew : the achievements of the biological sciences in the years 1980-1990, according to which genetic determinism dictates morphogenetic processes during animal and plant development. We now have a conceptual framework to explain how genetic activity - and the biochemistry that translates it into - enables the information needed to build a new organism to be transmitted from one generation to the next. For example, the information system that defines the spatial coordinates of each cell in a tissue or embryo. We have been able to build on this scientific heritage, but we have also realized that this genetic information, while important, is insufficient to account for the dynamics of shape acquisition. Firstly, our research has enabled us to understand that cellular mechanics are the source of the internal dynamics of a developing structure, whether an embryo or an organ. We have characterized the dynamic processes, and then tried to understand the mechanical processes that produce the forces necessary for the movements characteristic of the Dynamics of Living Systems. Since every movement has a cause, which is a force, we ask ourselves where this force comes from. Secondly, we have characterized the information that structures cell mechanics and the emergence of complex forms in space and time. This information is not strictly genetic.
The notion of biological information must therefore evolve ?
Yes, and today we're in the process of broadening this concept. Traditionally, if you ask any scientist what biological information is, they'll tell you that it's what prescribes the dynamics, and ultimately the form, of a living structure. In other words, genetic inheritance would inform and determine all cellular processes, establishing cell coordinates and prescribing movements in a deterministic fashion. But we want to redefine this conception of information to better understand the Dynamics of Living Systems. Firstly, by broadening its nature : it's not just genetics. Information is something that has the capacity to encode space and time ; the length of a biological tissue, or the number of cells that make it up. Today, we propose that information not only has a genetic and chemical component, but also a strictly mechanical and geometric one. Next, we characterize how this information is deployed, or flows. One might think that it flows according to an initial inherited plan, from top to bottom with a highly hierarchical structure and in a deterministic manner. However, we can also conceive of the flow of information within a self-organizing system, based on local stochastic fluctuations and feedback. A typical example of such a system is the anthill ; there is no general plan of organization, but local rules that enable the construction of a whole. We then want to understand how new information is produced. Every biological system relies on these two modes of information flow.
What are the potential applications (biological, medical...) of your research into the forces that govern the cohesion of the organism ?
Pathological processes can only be understood if normal physiological processes are also understood. Studies on development and morphogenesis have inspired greater consideration of the mechanical processes involved, particularly in cancer. Today, a whole field of biological investigation for therapeutic purposes consists in better understanding and taking advantage of organs' natural capacities for renewal and repair, notably through the activation of stem cells, in an attempt to repair anomalies linked to genetic lesions. We're talking here about synthetic biology ; we're trying to reconstitute an organ or an embryo in vitro. These approaches enable us to understand the information we need to give these cells to enable them to form a structure, and what they are capable of doing on their own through self-organization. Typically, we realize that they cannot reach their destination without the information they need to inherit. So, through a construction game, we provide them with what they lack to produce the physiological structure : this is a way of testing hypotheses about inherited information - the deterministic program - and about information newly produced by self-organization. It will be a question of a certain chemical cocktail, initial conditions favorable to their own differentiation, and a geometric and mechanical environment, which will favor or discourage their deployment. A whole branch of morphogenesis attempts to reproduce these forms in vitro in order to reimplant cultured organs in an organism and compensate for physiological dysfunction or repair tissue damage associated with aging.
You head the Turing Center for Living Systems, where biologists, physicists, computer scientists and mathematicians work together. Why is this interdisciplinary approach essential ?
Very early on, our own research led us to collaborate with physicists, as we were asking ourselves physics-related questions : what is the nature and origin of the forces at play in developmental processes ? We had to study the mechanical properties of the material to which the forces apply in order to understand the answers. A spring, for example, is elastic : you can deform it, but once the force has been removed, it returns to its original shape. A liquid, which is viscous, can flow, but once the force is removed, it doesn't return to its original shape. Biological materials are both elastic and viscous. Physicists are needed to characterize these physical processes, set up experiments and carry out measurements to obtain more quantitative data. As biological processes are highly complex, we can never be sure of knowing all the variables in a system ; in a watch or a car, there are a known number of parts, but in an organism, we don't know the functions of all the molecules. What's more, their interactions are often non-linear. When we establish a theoretical model, we describe precisely the rules of the system, its components, its interactions... So, we need predictive models, which will serve as a reference to know whether we understand a system correctly. We can then test whether the hypothesis accounts for the observed process. If the prediction doesn't work, we have to juggle between the experiment and the theoretical model, and that's why we need mathematical implementations and computer simulations. Gradually, by asking seemingly simple questions, we come to collaborate between biologists, physicists and computer scientists in the analysis of large-scale, highly complex data sets. Artificial intelligence and deep learning procedures are used to extract relevant information. Our research itinerary is fundamentally interdisciplinary, and this is increasingly the case on an international scale.
How did this interdisciplinarity come about in your research ?
This approach gained momentum when I spent a sabbatical year in India, where I collaborated with a theoretical physicist and a cell biologist. This experience led me to think about ways of building a research ecosystem in Marseille that would be more intrinsically multidisciplinary, and benefit all its players. In 2012, as part of the future investment program, and then again in 2016, I obtained substantial funding to set up this ecosystem with my colleagues and welcome non-biologists interested in biology. To do this, we first needed excellent biology, which attracts and inspires collaborators, and then calls for tender to recruit researchers in physics, computer science and mathematics. In ten years, one hundred and fifty to two hundred people have joined us ; PhD students, post-docs, engineers and permanent researchers. We now have a clear international profile and a remarkable diversity of research profiles. Secondly, we needed to facilitate collaborations ; every student recruited has at least two thesis or post-doctoral supervisors. As each collaboration allows us to recruit someone, this creates a network of interactions. Finally, we have set up interdisciplinary training programs : training biologists in physics, and vice versa, which is done within the framework of doctoral and masters programs. Our master's degree in computational biology and mathematics is a good illustration of this. Some of my lectures at the Collège de France form the basis of the lectures I give with a mathematician and a computer scientist in this master's program. The watchword is to create a place of interaction that encourages novelty. Interdisciplinarity is a driver of creativity.
Since 2017, you've held the Dynamics of Living Systems chair at the Collège de France. What do you draw from this experience ?
It's been an extraordinary experience for me. In particular, the lectures I give at the Collège enable me to put my interdisciplinary approach into practice. I explain physics to biologists and biology to physicists. My audience itself is built on this principle. In the final analysis, this fits in with my team's philosophy, and the efforts I make at the Collège go hand in hand with those I put into my day-to-day research and teaching work. After all, it's really when you're teaching that you check the solidity of what you've learned and understood. It also enables me to broaden the scope of my readings. For example, I was interested in questions of form, but also in controlling the size of structures. So, for two years, I devoted myself to this subject, which I didn't know very well, to build up a lecture on it, and in return, this enriched our own research with new directions directly inspired by my lectures. This approach anchored me all the more strongly to this notion of interdisciplinarity : teaching was what was most lacking in my career, and the Collège enabled me to discover it.
Interview by William Rowe-Pirra